MAVEN and the evolution of Mars
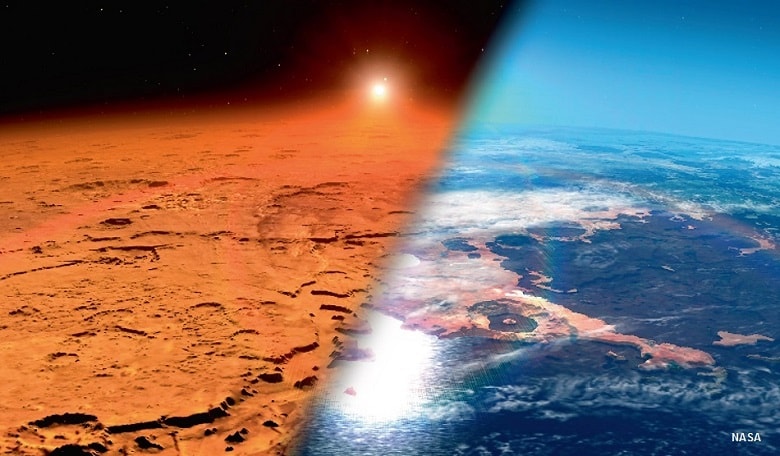
Janet G. Luhmann
Senior Fellow
If you already have a login and password to access www.room.eu.com - Please log in to be able to read all the articles of the site.